3. Disinfection by means of Ultraviolet Lamps
GENERAL
In practice, germicidal applications and design factors are governed by three main factors:
A.THE EFFECTIVE DOSE (Heff)
Effective dose is the product of time and effective irradiance (the irradiance that makes a germicidal contribution). However, dose is severely limited by its ability to penetrate a medium. Penetration is controlled by the absorption co-efficient; for solids total absorption takes place in the surface; for water, depending on the purity, several 10s of cm. or as little as a few microns can be penetrated before 90% absorption takes place.
B.THE POSSIBLE HAZARDOUS EFFECTS OF SUCH RADIATION
Germicidal radiation can produce conjunctivitis and erythema, therefore people should not be exposed to it at levels more than the maximum exposure given in Fig. 9. It follows that this needs to be taken into consideration when designing disinfection equipments.
Germicidal applications can be and are used for all three states of matter, viz. gases (air), liquids (mainly water) and solids (surfaces) with greatest technical success in those applications where the absorption coefficient is smallest.
However, some notable success has been achieved in applications where, despite a disadvantageous absorption, "thin film" or closed circuit (recycling the product) design techniques have provided effective solutions.
C. LAMPS
Five Philips ranges of lamps are available for disinfection purposes:
• Classic T5 and T8 TUV lamps
• High output TUV lamps
• PL-S and PL-L twin-tube compact TUV lamps
• and the newest addition: XPT amalgam “Very High Output” germicidal lamps in T6 and T10 diameters.
All of these are based on low-pressure mercury technology. Increasing the lamp current of low-pressure lamps produces higher outputs for lamps of the same length; but at the cost of UV efficiency
(UV watts/input watts); this is due to higher self-absorption levels, and temperature influences. The application of mercury amalgams, rather than pure mercury, in the lamps corrects for the latter.
• HOK lamps, which are of the medium pressure mercury type, mainly characterized by a much higher UV-C output than low pressure options, but at much lower efficacies.
The choice of the lamp type depends on the specific application. In most cases the low-pressure types are the most attractive. This is because germicidal lamps are highly efficient in destroying micro-organisms, hence there is limited need for high wattage lamps. For water disinfection, low and medium-pressure are both used, although the choice is not necessarily based on UV-C efficacy. Initial total systems costs, including metalwork and space limitations, can be the driving factor rather than efficacy.
3.1 AIR DISINFECTION (Ref.12, 13)
Good results are obtained with this form of disinfection because air has a low absorption coefficient and hence allows UV-C to attack micro-organisms present. In addition, two other beneficial conditions are generally present, viz. random movements allowing bacteria etc. to provide favorable molecular orientations for attack and high chances of "closed circuit" conditions, that is second, third and more recycle opportunities. From this, it is evident that air disinfection is an important application for UV radiation.
Even in the simplest system (natural circulation) there is an appreciable reduction in the number of airborne organisms in a room. Thus the danger of airborne infection, a factor in many illnesses, is considerably reduced.
However, it should be remembered that disinfected air is not, in itself, a disinfecting agent.
Presently, there are five basic methods of air disinfection using ultraviolet lamps viz:
a. Ceiling or wall mounted TUV lamps
b. TUV lamps (in upwards-facing reflectors) for upper-air irradiation.
c. TUV lamps (in downwards-facing reflectors) for irradiation of the floor zone (often in combination with b.).
d. TUV lamps in air ducts sometimes in combination with special dust filters.
e. TUV lamps, incorporated in stand alone air cleaners with a simple filter.
3.1.1 CEILING-MOUNTED TUV LAMPS
This method is used in those cases where either the interior is unoccupied or where it is possible for the occupants to take protective measures against radiation. These protective measures entail covering the:
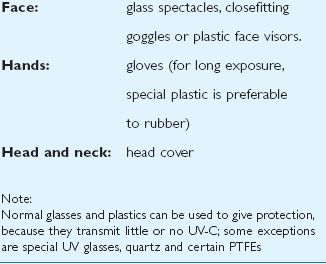
3.1.2 TUV LAMPS FOR UPPER-AIR IRRADIATION USING UPWARD FACING REFLECTORS
This method of disinfection can be used to combat bacteria and moulds; it also has the advantage that it can be used in occupied interiors without the occupants using protective clothing. The lamps should be mounted in suitable reflectors and aimed to emit no radiation below the horizontal.
The reflectors should be mounted more than 2.10m above the floor, the lower air is thus entirely free of any direct ultraviolet radiation. Air above the 2.10m level maintains a low germ level, because it is subject to direct UV-C radiation.
Free convection of air without forced ventilation causes air movements of about 1.5 - 8 m3 per minute, thus producing exchanges between the upper treated and lower untreated parts of the room. The process reduces air contamination to fractions of that before the TUV lamps were activated. As an indication for general applications in a simple room, or enclosure, it is advisable to install an effective UV-C level of:
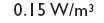
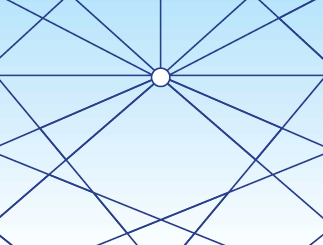
Fig 15.Various principles of air disinfections
a. Ceiling mounted lamps.
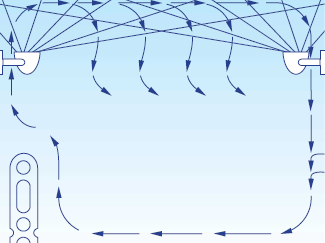
b. Upwards facing reflectors.
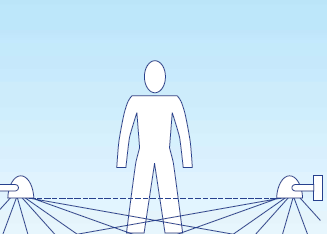
c. Downwards facing reflectors.
3.1.3 TUV LAMPS FOR IRRADIATION OF THE FLOOR ZONE USING DOWNWARD FACING REFLECTORS
This method is for use in those cases where it is important that the entire room air, even at floor level is rendered as sanitary as possible. In this case, lamps supplementing those irradiating the upper air should be fitted in downward-aimed reflectors at about 60 cm above the floor.
In methods 3.1.1, 3.1.2 and 3.1.3 person detectors/systems can be used to deactivate TUV lamps, if necessary.
3.1.4 TUV LAMPS IN AIR DUCTS
In this method, all the conditioned air is subjected to radiation prior to entry. The injected air can be disinfected to a specified killing level, depending upon the number of lamps installed and the dwell time, that is the time spent in the effective killing region of the lamp(s); by definition this takes the dimensions of the air duct into consideration.
Such systems have a controlled flow rate and their performance can be predicted theoretically.
Certain aspects should be borne in mind, however
• These installations are only suitable for
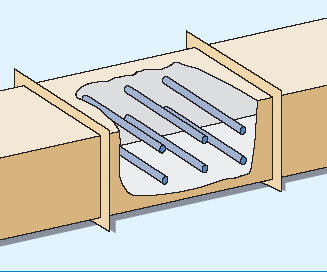
Fig 16. Basic arrangement of TUV lamps in an air duct for room disinfection.
bacteria; most moulds have higher resistances to ultraviolet, so the air flow rate is not likely to allow a sufficient dwell time to produce a high enough effective dose.
• Dust filters should be installed to prevent the lamps from becoming soiled and hence seriously reducing their effective emission.
• The number of lamps required in an air disinfecting chamber in an air duct system is dependent on the required degree of disinfection, the airflow rate, the ambient temperature, the humidity of the air and the UV-reflecting properties of the chamber walls.
The advantage of disinfecting air prior to it entering a room is that there is then no limit to the maximum permitted radiation dose, since humans are totally shielded.
Designing duct systems needs to account for practical issues, such as large temperature and humidity variations caused by exterior weather variations, if only because air is often drawn from outside, then released into a room after a single pass over the lamps. Recycling part of the air will allow multiple passes, hence improving system efficiency.
Lining the UV lamps section with aluminium, also increases efficiency. The lamps and the wall of the duct should be easily accessible to permit regular cleaning and easy maintenance, another reason for a modular design.
Micro-organisms exposed to UV, experience a normal exponential decrease in population,
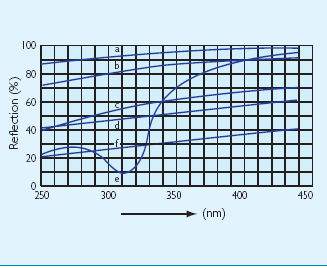
Fig 17. Metal surfaces. a. Evaporated aluminium d. Nickel b.Aluminium foil e. Silver c. Chromium f. Stainless steel
as already expressed on page 10:

The rate constant defines the sensitivity of a microorganism to UV radiation and is unique to each microbial species. Few airborne rate constants are known with absolute certainty.
In water based systems, Escherichia coli are often used as test organism. It is however not an airborne pathogen. For aerosolization tests, often the innocuous Serratia marcescens is used.
POINTS TO REMEMBER WHEN CONSTRUCTING TUV LAMP INSTALLATIONS IN AIR DUCTS:
• The surface of the chamber walls should have a high reflectance to UV 254 nm, for example by using anodized aluminium sheet (reflectance 60-90 per cent).
• The lamps should be so arranged that there are no ‘shadow’ areas.
• Lamps should be mounted perpendicular to the direction of the airflow.
• Lamps and the inner (reflecting) walls of the chamber should be cleaned frequently using a soft cloth.
• Lamps should be changed after the nominal lifetime; an elapsed time meter will help.
• An external pilot light should be used to indicate that the lamps are functioning.
REFLECTANCE OF VARIOUS MATERIALS TO UV 254 NM
The graphs shown across give the spectral reflectance of various metals (Fig. 17) and organic substances (Fig. 18) to radiation of different wavelengths. These graphs demonstrate the importance of determining a material’s 254 nm reflectance. As can be seen, high reflectance to visible radiation is not consistent with high reflectance to short-wave ultraviolet radiation.
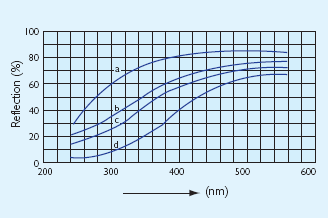
Fig 18. Organic substances a. Bleached cotton c. Linen b. White paper d. White wool
Materials with a high reflectance to 254 nm are used to construct reflectors for both direct and upper-air irradiation. Materials with a low reflectance to 254 nm are used where ultraviolet radiation has to be absorbed after performing its function.
This latter is necessary to avoid the consequences resulting from the unwanted 254 nm reflections, so ceilings and walls should be treated with a low reflectance material for people comfort and safety factors.
3.1.5 TUV LAMPS IN STAND-ALONE UNITS
Recently this method has gained commercial favor by meeting a growing need for a better indoor air quality.
Closed stand- alone devices are safe, simple and flexible. In essence the units consist of TUV lamps, mostly PL-L types driven by high frequency ballasts, mounted inside a "light trap" container. The unit incorporates a fan that firstly draws air across a filter, then across the lamp(s). Single and multiple lamp options can be built into a small outer using either single or double-ended lamp options. For maximum design flexibility, PL-L and PL-S lamps offer the best solutions, because their dimensions are compact, so reducing unit size and because their single ended configuration allows more mounting options.
The units have the benefits of portability and hence more mounting positions viz. wall, floor or ceiling mounted in either permanent or temporary options. A feature of their design is that cleaning and lamp and filter replacement is easy.
Additionally their portability can be used to produce immediate results. Variation in UV-C dose can be achieved both by varying the number of lamps and their wattage (see also dimming below). As an example, it is possible to use the same physical design dimensions for PL-L lamps with a nominal wattage range between 18 and 95W “HO”,
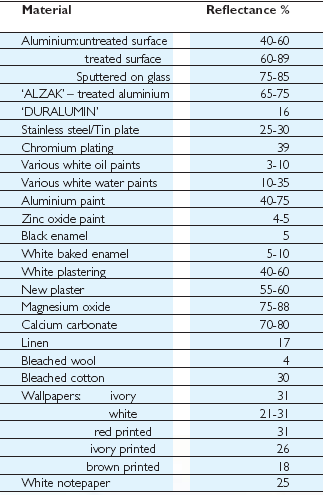
Table 3. Reflectance of various materials to UV-254 nm radiation.
in single and multi lamp variants. Commercial products are known for as few as 1 x PL-L 18W and as many as 4 x PL-L 95W HO lamps inside the same container, giving a unit capable of producing a 25-fold difference in effective dose.
PL-L lamps are more flexible; they have readily available and competitively priced electronic regulating (dimming) ballasts to vary UV output in a simple reliable fashion. Ballasts can be single, double and in the case of 18W, four lamp versions. This adds to the flexibility of portable units.
3.2 SURFACE DISINFECTION
Surface disinfection generally requires high-intensity short-wave ultraviolet radiation. Mostly this means TUV lamps are mounted close to the surface requiring to be kept free from infection or to be disinfected.
The success of surface disinfection depends largely on the surface irregularity of the material to be disinfected, because UV radiation can only inactivate those micro-organisms that it hits with a sufficient dose. Thus disinfection can only be successful if the entire surface is exposed to UV radiation. Micro-organisms sitting in "holes" in a surface are not likely be overcome by reflections from the hole walls, as can be deduced from the reflectance shown in table 3.
In practice, solid surfaces, granular material and packaging (whether plastic, glass, metal, cardboard, foil, etc.) are disinfected or maintained germ-free by means of intensive, direct irradiation. Additionally, disinfected material can be kept largely germ-fee throughout its further processing by irradiating the air along its path.
3.3 LIQUID DISINFECTION
Germicidal energy radiation is capable of penetrating liquids with varying degrees of efficiency. From a treatment view, liquids can be regarded as similar to air so the further the UV radiation is able to penetrate the liquid, the more efficient is its action. The degree of efficiency thus greatly depends on the liquid and more particularly its absorption coefficient at 254 nm (table 4). As an example, natural water’s transparency to 254 nm may vary by as much as a factor of 10 or more from place to place. Polluted industrial water often needs purification followed by disinfection; here UV-C is growing with many thousands of systems in use in North America and Europe, each with a multitude of lamps. Often UV radiation may supplement or replace conventional chlorination measures (see later).
UV-C has advantages over chlorinating techniques, because it produces far fewer noxious by-products and is it unaffected by the pH of the water or its temperature. The reader should note that the latter comment refers to the radiation, not to the lamp, or its environment as described earlier.
Micro-organisms are far more difficult to kill in humid air, or in a liquid environment, than in dry air. This is because they limit transmission of 254 nm radiation. In more quantitative terms liquids decrease the germicidal intensity
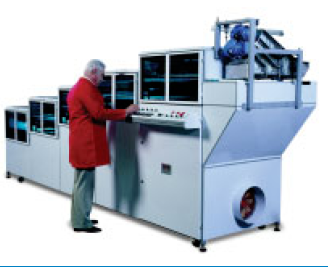
Fig 19. UV “cascade” surface disinfection of spices.
exponentially according to the formula
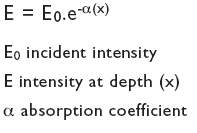
Liquids with a high ά can only be disinfected when they are exposed as thin films. A rough indication to estimate penetration depth is 1/ά, at this depth the irradiation level will have fallen to 1/e or to 37%.
To overcome wall effects where liquids are notoriously static, turbulence or rigorous stirring is necessary for better disinfection, agitation helps orientate micro-organisms hidden behind particles.
Iron salts (as well as other inorganic salts) and suspended matter in liquids will decrease the effectiveness of germicidal radiation.
Additionally, it is feasible that organic compounds, in particular, those susceptible to bond fissure under UV radiation, can change the texture and taste of the liquid being treated.
Hence experimentation is needed. In round terms the effective depth of penetration for a 90% kill may thus vary from 3m for distilled water, down to 12cm for normal drinking water and even less in wines and syrups (2.5mm) See table 4.
The penetration depths cause more special techniques to be applied to allow 254 nm radiation to penetrate sufficiently, these include generating "thin films" and or slow speed presentation to the radiation, so that a sufficient dose can be applied.
If an UV lamp has to be immersed in a liquid, it should be enclosed in a quartz or UV-C transparent PTFE sleeve.
Installations for disinfecting liquids may have the following forms:
1. One or more lamps enclosed in a quartz container or one of similar material (with a high transmittance at 254 nm), which is surrounded by the liquid to be disinfected. A multiple of such configurations can be used inside one outer container.
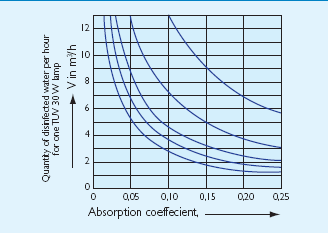
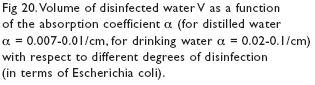
2. A quartz tube (with high transmittance
at 254 nm) transporting liquid surrounded by a cluster of lamps in reflectors or by an integral reflector TUV lamp e.g. TUV115W VHO-R.
3. Irradiation by means of lamps installed in reflectors or integral reflector TUV lamps e.g. TUV115W VHO-R mounted above the surface of the liquid.
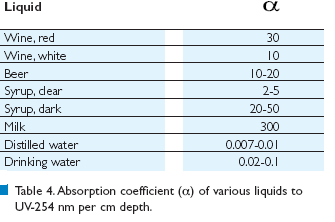
4 Applications
GENERAL
The main application areas for UV germicidal lamps may be briefly classified below, although there are many other areas, where the lamps may be employed for various purposes.
• Air disinfection
• HVAC cooling coils
• Residential drinking water
• Industrial drinking water
• Process water (beverages)
• Waste water
• Semiconductor and IC manufacture
• Pools, spas, aquaria, fish ponds.
4.1 AIR DISINFECTION
Indoor air is trapped, often re-circulated and always full of contaminants such as bacteria, viruses, moulds, mildew, pollen, smoke and toxic gasses from building materials.
Increasing levels of such contaminants act as triggering mechanisms for a variation of diseases of which asthma is the most prominent.
For offices and in industrial environments, so called HEPA (High Efficiency Particulate Air) filters are installed in HVAC ductwork. Very fine fibres, pressed together, form a structure with openings, too small for most particulate contaminants. Such filters are effective, but always will give rise to considerable drop in air pressure. In recent days, growing concern
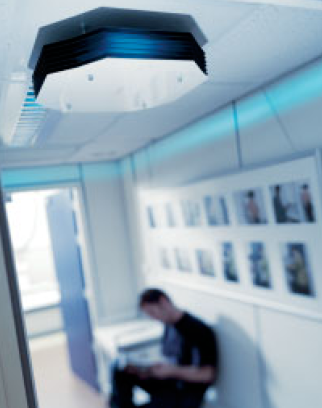
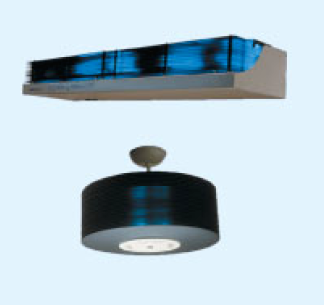
for indoor air quality has lead to new measures. Application of UV in air ducts for ventilation, heating and cooling purposes has proven to provide adequate protection against airborne pathogens.
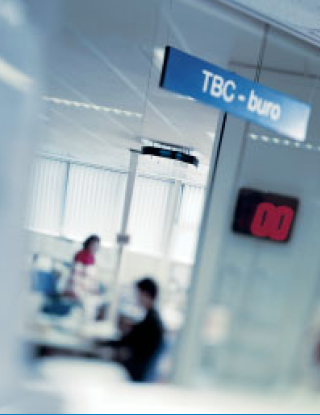
For domestic use some very different basic types can be considered:
• Fiber mesh filters, generally designed for a particle size of 25 microns or larger.
• Activated carbon filters, which will neutralize some gasses, smoke and odors.
• Electronic air cleaners, which charge particles such as dust, pollen and hair. The charged materials are attracted by a series of opposite polarity charged metal plates.
• Ozone and ion generators
• UV light, the only treatment, truly lethal to micro-organisms
With patients and visitors bringing in pathogens that cause diseases such as tuberculosis, wards, clinics, waiting and
operation rooms and similar areas should be protected against the risk of infection in personnel and patient populations, if possible at a reasonable cost! Common traditional disease controlling methods in hospitals are:
• Ventilation: dilution of potentially contaminated air with uncontaminated air
• Negative pressure isolation rooms
• HEPA (High Efficiency Particulate Air) filtration
UV germicidal irradiation provides a potent, cost effective solution to upgrade protection against infection. (Ref. 12,13)
Especially, upper-air disinfection has proven to be very effective to supplement existing controls for TB and other airborne diseases (Ref. 8). Many disease-causing organisms circulate on air currents in "droplet nuclei", 1 to 5 micron in size, that are expelled with a cough, sneeze or even with speech. These droplet nuclei can be inhaled, spreading infections.
It is estimated that up to 99% of airborne pathogens are destroyed with adequate air circulation and UV exposure.
4.2 COOLING COILS
Air conditioner cooling coils are almost always wet and dusty and thus can serve as an ideal breeding ground for moulds, a known allergen. Coil irradiation with UV drastically reduces or prohibits growth of moulds. At the same time heat exchange efficiency is improved and pressure drops decrease. As the coils are constantly irradiated, only a modest UV irradiance is required.
4.3 WATER PURIFICATION (Ref. 7, 14)
A wide variety of micro-organisms in the water can cause disease, especially for young and senior people, who may have weaker immune systems.
UV light provides disinfection without the addition of chemicals that can produce harmful by-products and add unpleasant taste to water. Additional benefits include easy installation, low maintenance and minimal space requirements.
UV has the ability to inactivate bacteria, viruses and protozoa. Each type of organism requires a specific dose for inactivation. Viruses require higher doses than bacteria and protozoa. Understanding the organisms to be neutralized will help to determine to size of the UV system that will be required. For example, to kill 99,9% of E.coli, a UV dose of 90 J/m2 or 9 mW.sec/cm2 is required.
UV installations are suitable for industrial, commercial and residential markets.
The quality of the water has an important effect on the performance of UV systems. The common factors that have to be considered are iron, hardness, the total concentration of suspended solids and the ultraviolet transmittance. Various organic and inorganic compounds can absorb UV.
When there is uncertainty about what may be present in the water, the UV transmittance should be tested. Most drinking water supplies have UV’ transmittances between 85% and 95%.
Separate treatment technologies often are required to improve the water quality before disinfection:
• Sediment filters, to remove particles that "shadow" microbes or absorb UV
• Carbon filters, which remove organic compounds and undesirable odors.
• Water softeners to reduce hardness.
UV is often used in conjunction with Reverse Osmosis (RO) applications. Disinfection prior to the RO systems increases the durability of the RO membrane by reducing the accumulation of bacterial biofilms.
The reactor of a UV disinfection device must be designed to ensure that all microbes receive sufficient exposure of the UV.
Most manufacturers of UV equipment use low-pressure mercury lamps. High output (HO) versions are rapidly becoming popular. High capacity drinking water and waste water systems feature medium pressure mercury technology.
The temperature of the lamp surface is one of the most critical factors for UV reactor design. The UV efficiency of the lamp (UV output per consumed electrical Wattage) strongly depends on the bulb temperature. (See Fig.28)
The diameter of the protective quartz sleeve should be carefully adapted to the specific power of the lamp (Watts per unit of arc length), as well as temperature and velocity of the water flow.
As the lamp ages, the UV output declines due to solarization of the lamp (glass or quartz) envelope. The quoted dose for a specific unit is the minimum dose that will be delivered at the end of the lamp’s life.
Most manufacturers offer electronic power supplies, that are more efficient (up to 10%) and operate at lower temperatures. Such ballasts normally withstand wide fluctuations in supply voltage, still providing a consistent current to the lamps.
Factors, that should be considered, when, choosing the right size of UV equipment, in order, to achieve the desired disinfection objectives are peak flow rate, the required dose and the UV transmittance of the water.
Theoretical calculations should be validated by bioassay tests, for a variety of conditions that include flow rates and variable water quality.
4.3.1 RESIDENTIAL DRINKING WATER
Classic Point of Use (POU) / or Point of- Entry (POE) UV disinfection systems consist of a low-pressure mercury UV lamp, protected against the water by a quartz sleeve, centred into a stainless steel reactor vessel.
The UV output is monitored by an appropriate UV sensor, providing visual or audible indicators of the UV lamp status. To improve taste and odor of the water POU systems are often used in conjunction with an active carbon filter.
The new ANSI/NSF Standard 55 (Ultraviolet Microbiological Water Treatment Systems) establishes the minimum requirements a manufacturer will need to become certified for a Class A or B UV system.
Class A POU/POE devices are designed to disinfect micro-organisms, including bacteria and viruses, from contaminated water to a safe level. Waste water is specifically excluded from being used as feed-water. As of March 2002 the UV system has to produce a UV dose of 40 mJ/cm².
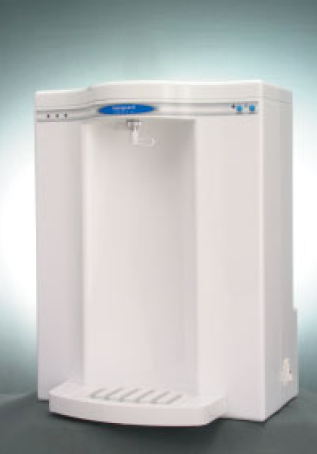
Fig 21. POU residential drinking water UV Disinfection device.
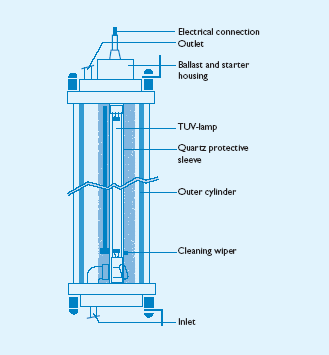
Fig 22. Basic sketch of TUV lamp operated water-disinfecting unit for general use.
Class A devices are required to have a UV sensor, alarming when the proper dose is not reaching the water.
Class B POU systems are designed for supplemental bacterial treatment of treated and disinfected public drinking water. Such devices are not intended for disinfection of microbiologically unsafe water. The systems are capable of delivering a UV dose of at least 16 mJ/cm2 at 70% of the normal UV output or alarm set point.
The 2002 version of Standard 55 clarifies all requirements for component certification. For instance, a 15-minute hydrostatic pressure test is needed.
4.3.2 INDUSTRIAL (MUNICIPAL) DRINKING WATER
Disinfection of drinking water by UV light is a well-established technology in Europe. Hundreds of European public water suppliers have by now incorporated UV disinfection. The driving force in Europe was to inactivate bacteria and viruses, but avoid use of chlorine. Recent studies regarding potential negative health effects of disinfection byproducts have led to a critical view on chlorine.
A few fatal waterborne outbreaks of cryptosporidiosis in North America have proven the fact that existing disinfection and filtration technologies could not guarantee to eliminate cryptosporidium oocysts from the water.
Cryptosporidium parvum is a human pathogen, capable of causing diarrhoeal infections, sometimes even leading to death. The organism can be shed as an environmentally resistant form (oocyst) and persists for months.
Cryptosporidium is almost completely resistant against chlorine. Ozone can be effective, but the water quality and temperature play a significant role. Its small size makes it difficult to remove by standard filter techniques.

Fig 23. UV drinking water plant 405.000 m³ per day, Tollyaytti (Russia)
Recent studies have verified that UV can achieve significant inactivation of cryptosporidium at very modest doses. Exposures as low as 10 mJ /cm2 will result in a more than 4- log reduction of concentration.
The effectiveness of UV for cryptosporidium removal, together with stricter limits on disinfection by-products will pave the way for UV disinfection in North America.
Due to their high UV efficiency, low-pressure high-output lamps will certainly find their way in many municipal UV drinking water facilities. However, as space always will be a problem, the high intensity medium pressure lamps will be favorite, especially when existing drinking water plants have to be upgraded with a UV extension.
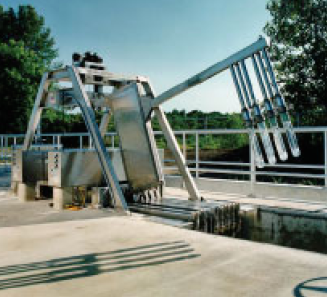
Fig 24. Waste water system.
4.3.3.WASTE WATER
Chlorine has been used to disinfect waste water for over a century. However, while chlorine is very effective, it is also associated with environmental problems and health effects. Chlorination by-products in waste water effluents are toxic to aquatic organisms, living in surface waters. Chlorine gas is hazardous to human beings.
UV irradiance has proven to be an environmentally responsible, convenient and cost-effective way to disinfect public waste water discharges. UV disinfection is much safer than waste water systems that rely on chlorine gas, as it eliminates transport and handling of large quantities of this hazardous chemical. More than 2000 waste water installations all over the
world rely on UV disinfection these days. The required UV dose levels depend on the upstream processes, and range, taking into account flow rates and UV transmittance of the water, between 50 and 100 m J/cm².
4.3.4. WATER COOLERS, DISPENSERS
Water vending machines store and dispense water that is non-chlorinated. The machines must be licensed by local health service departments. One of the requirements for the license is that the vending machine is equipped with a disinfection unit to reduce the number of bacteria and other micro-organisms.
Bottled water coolers, which also dispense non-chlorinated water, are not required to contain a disinfection unit.
However, without an active disinfection system, also bottled water cooler reservoirs are subject to biofilm growth. Such biofilms act like a breeding place for bacteria, protected by the gel-like substance. Bacteria contamination, regardless of whether it is non-harmful or even beneficial, is not a quality to be associated with drinking water. To avoid biofilm growth often simple UV reactors are being introduced.
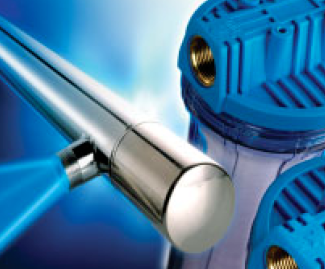
4.3.5 COOLING TOWERS
Cooling towers and re-circulating loops are often dir ty, warm and rich in bio-nutrients. They are perfect breeding places for micro-organisms.
Chemical compounds, like chlorine or ozone, are fed into the system in regular intervals, to control the rate of biological growth. UV will substantially decrease the costs of disinfection, without any safety or environmental issues.
4.3.6 SEMICONDUCTORS
PROCESS WATER
Organic compounds, present in the rinse water, can affect production yields and product quality in the semiconductor industry. The total organic carbon (TOC) contamination level is specified to be less than one part per billion (ppb) for
ultra pure water, used for this application. Ultraviolet light represents a powerful technology that has been successfully introduced in the production of ultra pure water for semiconductor, pharmaceutical, cosmetics and healthcare industries. Its powerful energies can be applied, not only for disinfection, but also TOC reduction and destruction of ozone and chlorine.
Two different UV wavelengths are employed, 254 nm and 185 nm. The 254 nm energy is used for disinfection. It can also destroy residual ozone, present in the water.
The 185 nm radiation decomposes the molecules. It carries more energy than the 254 nm and is able to generate hydroxyl free radicals from water molecules. These hydroxyl radicals are responsible for oxidizing the organics to carbon dioxide and water molecules.
185 nm radiating lamps are made of special quartz, with high transmittance for the lower wavelengths. Typical dosage requirements range from 100 to 500 mJ/cm2. Philips XPT amalgam lamps in a 185 nm version, but also HOK and HTK medium pressure lamps can provide excellent solutions.
4.3.7 SPAS AND SWIMMING POOLS
TUV lamps are used to supplement the traditional methods of water treatment.
Importantly, with UV-C as a supplement, chlorination methods need less chlorine for the same result. This is welcome both for those with allergies and those with distaste for chlorine.
The reason that UV-C is not suitable for sole use is that swimming pool water circulation has to take into consideration solids, inorganic compounds, hence filtration and chemical processes are also needed. A standard technique is to circulate part of the water through a continuous flow UVC device, thus creating a partial closed loop system; this in tandem with the chlorinator produces effective disinfection. It can lower the chlorine dose up to 50%.
4.4 REDUCTION OF ALGAE IN
FISH PONDS
Fishponds owners are often troubled by phototrophic micro-organisms. These are typical water organisms widely distributed in both fresh and salt water. Phototrophic bacteria contain photosynthetic pigment and hence they are strongly colored and appear as dense suspensions of either green, olive, purple-violet, red, salmon or brown. Seasonal effects may lead to massive growth (‘flowering of the water’) as light helps chlorophyll synthesis.
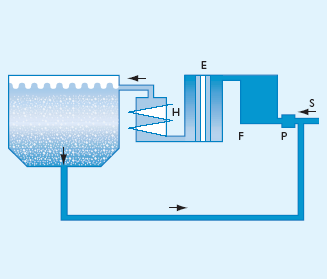
Fig 25. Schematic representation of a water purification system for a private swimming pool E=U.V. radiator F=filter H=heating P=pump S=fresh water supply.
If algae are to be destroyed or their growth inhibited, either a high dose of UV 254 nm radiation is needed or a long irradiation time. These conditions can be met relatively easily by creating a closed loop system whereby the water is presented to the UV-C source a number of times per day. The lamp is encased in a quartz tube. In practice, it has been found that, for instance, a TUV PL-S 5W lamp in series with a filter can keep a 4.5K liter (1,000 UK gallons) pond clear. For larger pond or pool volumes higher output lamps are needed on a pro rata scale. The process is thought to be that algae are split, recombine to form larger molecular chains, which can be removed by the filter, or are so large that they sink to the bottom of the pond.